Spaceships: something's gotta make them go. Even if you have made up a nice way of going faster than light -- wormholes, warpdrives, hyperspace, improbability drives -- there are going to be bits when your characters have to travel more mundanely. You can't go into orbit when you're travelling faster than light. Unless you happen to be inside the event horizon of a black hole, but then you have other problems.
This post is about relatively non-relativistic (yeah, I did that on purpose, so shoot me) methods of propulsion. To be used in local space only (or if you want to throw your characters into suspended animation or trap them on a generation ship, or something).
Rockets!
OK, it's pretty much mandatory that
I start with rockets since they are what got us into space in the first
place. Specifically chemical rockets, since the term has come to be
used more broadly in some circles.
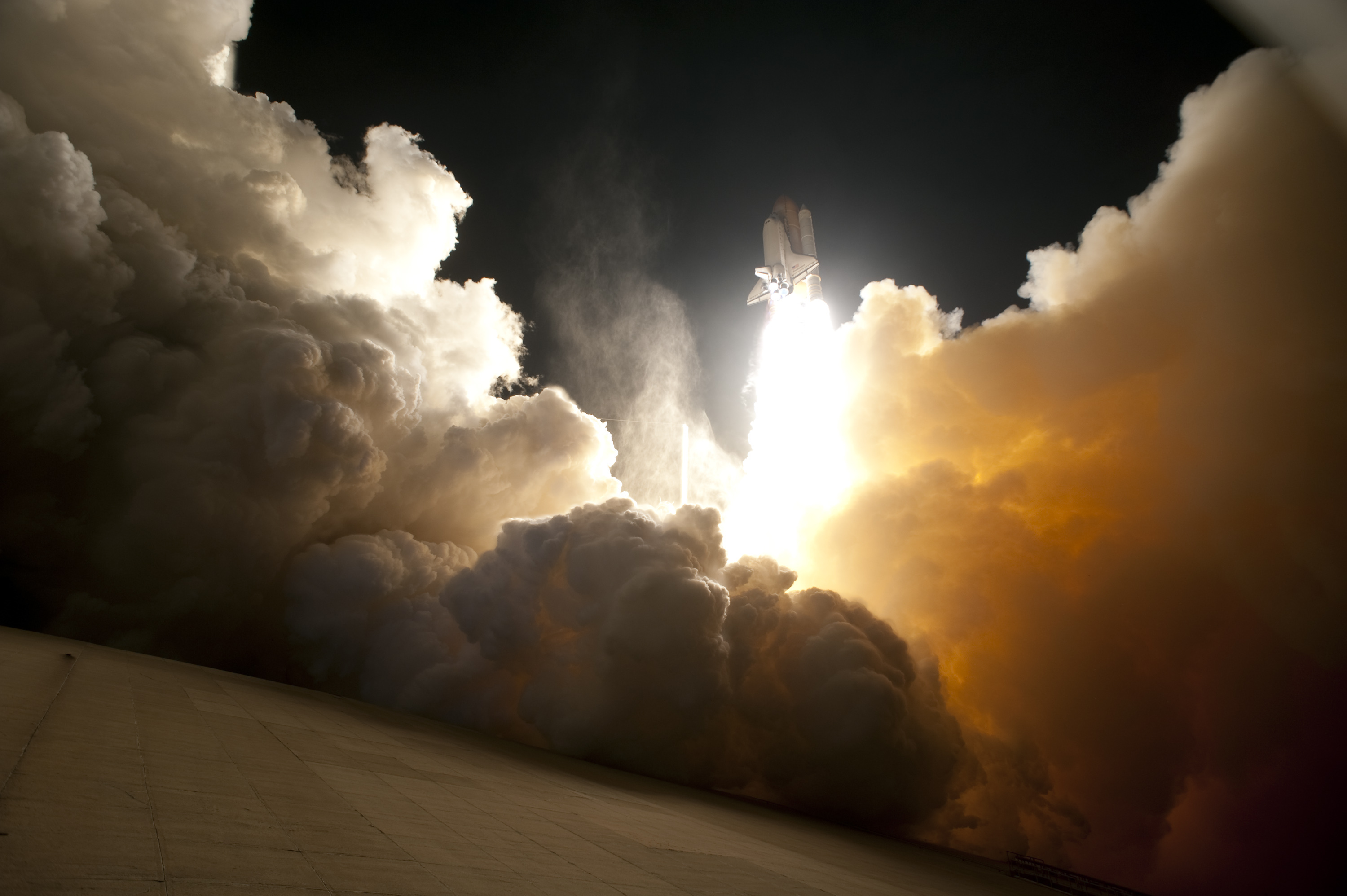 |
Space shuttle Endeavour taking off.
Credit: NASA |
The basic premise is: set things on fire explosively, direct the explosion away from where you want to go, and conservation of momentum does the rest. Why conservation of momentum? In the greater scheme of the universe, momentum must be conserved. It cannot be created spontaneously and it cannot be destroyed. It's one of those immutable laws. So, the
only way to move is by exchanging momentum with something else. Say you sit on a particularly good wheeled chair. If you throw your heavy, laptop-filled bag away in front of you, the chair will move backwards a bit. Assuming you were stationary to begin with, when you threw the bag, you imparted it with momentum. (Momentum, by the way, is just the product of mass and speed, nothing
too mysterious.) But momentum has to be conserved, so to compensate, you and your chair moved backwards with momentum exactly equal and in the opposite direction to that of the bag. Of course, this example isn't perfect since the wheels of the chair are also slowed down through friction, but ideally, your mass plus the chairs mass, multiplied by how quickly you moved backwards, would be equal to the mass of the bag multiplied by the speed at which you threw it.
In a frictionless environment, such as space, throwing something as small as an apple (also, congrats on smuggling an apple onto your space station) would propel you backwards noticeably. This is what most fuels do, in essence. They throw something backwards as hard as they can so that they can go forwards.
Commonly, rockets (that take off from Earth) have two tanks, one filled with liquid oxygen and one with liquid hydrogen. When combined they react very quickly and exothermically* which leads to a rapid release of energy and water. Because water is what you get when you put a lot of hydrogen near a lot of oxygen. (Really, putting a lot of hydrogen near a little bit of oxygen also ends
explosively.) And water is what you get when you burn hydrogen (and if you're wondering "burning" something is just a colloquial way of saying "oxidise rapidly" or "react with oxygen"). The good news is that launching rockets doesn't have a large carbon footprint (because no carbon is involved in the actual launch process); building them is another matter.
Back to the point, oxygen/hydrogen are used as propellants because they give off the most energy of any practical combination of chemicals. Somewhere, probably on the internet, I once came across a table of effectiveness of different fuels. I would love to link to it now, but that was several years ago and I could not find it again. The closest I got was
this table in Wiki, which is more a table of different propulsion methods.
* Exothermic reactions are reactions that release energy. Conversely, endothermic reactions consume energy.
Ion drives
Ion drives are a term that gets bandied around in both science fiction and "future of science" type discussions every now and then. There are actually a few different ways to build an ion drive (see aforementioned table in Wiki for details). The basic premise is that instead of using a chemical reaction to push something out the back of the spacecraft, you use electric fields to push ions (atoms with either a positive or negative charge; positive one are being pushed out, usually) away from the spacecraft. (Which you might have ionised with a laser or similar.)
The main downside is that the accelerations generated are fairly low, so it would take a long time to get anywhere. Unlike chemical rockets, however, ion drives would generally take a lot longer to run out of fuel. It might also be possible to scoop up stray atoms from space, ionise those and use them as propellant (this part, completely untested right now; ion drives in principle have been constructed though). So, although you start off slowly, if you keep accelerating constantly over a long period of time, the ion drive could leave you with considerable speed by the time you arrive. On the other hand, now that I think about it, they probably wouldn't work for decelerating suddenly, nor for manoeuvring.
Really, ion drives are just another way of throwing things backwards to make you go faster.
To read more, go look at the
NASA Deep Space 1 page, a mission powered by an ion drive.
Solar sails
Solar sails, on the other hand, work on a slightly different principle. Instead of bringing fuel along on the trip and throwing it out, a solar sail works, well, similarly to a sail on a boat. You throw your fuel at it to make it go forwards.
Just as wind pushes boats along by the sails, so does light push solar sails along.
Light can't push things! I hear you exclaim. (Or is that the sleep deprivation talking?) Actually, yes it can. Photons, particles of light, have a momentum associated with them. It is calculated a little differently to momentum for objects/particles which
do have mass; instead of mass times velocity it's Planck's constant divided by wavelength (exactly why is a bit complicated. Quantum is involved).
So a solar sail works by "collecting" the momentum of the light that hits it. The source of that light could be the sun or it could be a giant laser. The advantage of the sun is that it's free, but the disadvantage is that sunlight is a little dilute from an Earth's distance away so the acceleration would be fairly slow. A laser would start off more concentrated and impart a lot more initial acceleration, but once the sail was out of range you'd be left with a giant laser and nothing to point it at. (I suppose some people wouldn't see this as a disadvantage.) Perhaps some combination of the two would be best, but in the real world, we're not up to that sort of testing.
Below is a picture (CGI sadly. I so wanted this to be a photo taken from the ISS or something) of NASA's NanoSail-D which they recently deployed. From memory, there was a bit of trouble getting the thing to unfurl properly (definitely can't launch with those sails fully extended) and then after a few days it magically worked.
Here is more info from NASA.
So there you have it, a few ways for your characters to travel mundanely, mainly within a system.